Surface Plasmon Resonance
The past articles have covered some of the most common examples of label-based technologies (see our previous article – Practical Examples of Biosensors); in the following we discuss one of the most popular label-free technologies on the market.
What is SPR?
Surface Plasmon Resonance (SPR) is an optical biosensing technology that allows the detection of molecular interactions. Although it is the name of the technology, it is also the name of the phenomenon behind the technology. SPR is an established technology (35 years) to detect analytes in solution with very high sensitivity.
How does it work?
The SPR phenomenon occurs when transverse magnetic (TM) polarized light strikes an interface between a metal and a dielectric material such as glass. When light travels from a medium with a higher refractive index (n1) to a medium with a lower refractive index (n2), it can be totally reflected, as shown in the figure below. This happens at any angle θ above a certain threshold angle, which can be calculated.

How can we detect and quantify molecular interactions with this setup?
We start by covering a gold film with a repellent surface coating made of proteins. We then embed ligands specific to the analyte of interest (recognition molecules) within this coating (gold film). This coated gold film is placed onto a glass layer, with the coating facing upwards. The sample solution that may or may not contain the analyte of interest (mobile phase) flows over this coated surface. If the sample contains the analyte, it will bind to its specific ligands on the surface. When we shine polarized light onto this surface at a specific angle θ, a new wave (called “mode”) is excited: a ). Because energy is required for these waves to form, a part of the energy normally redirected into the reflected light wave is used to create these waves. At this specific angle θ, where the surface plasmon is formed, all the light energy goes into forming the SP mode; the light is virtually entirely extinguished.
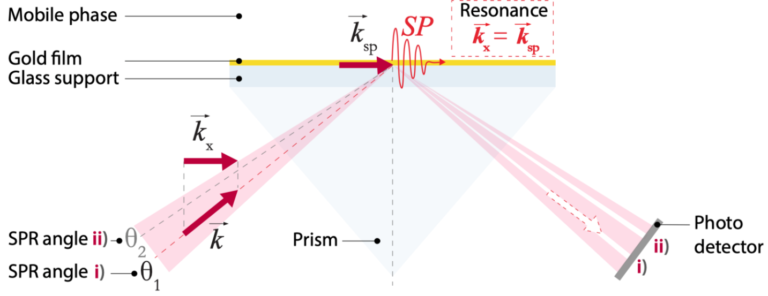
When preparing the surface plasmon resonance biosensor, the specific angle θ where this effect occurs is established. It is crucial for this angle to be measured, because whenever a molecule binds to the recognition molecules in the surface coating on the gold film, the angle at which the SP mode is formed slightly changes. Indeed, when a molecule binds to the recognition molecules in the surface coating, the refractive index close to the surface is altered, which in turn alters the angle at which the plasmon is launched.
By letting the sample of interest flow over the coated gold film, we can measure the angle dependent intensity of the reflected light beam with a photodetector array, as shown in the figure below. This angle-dependent light intensity is proportional to the refractive index close to the surface, which is itself proportional to the amount of analyte binding: by using the angle shift in the reflected light beam, we can indirectly quantify the amount of analyte binding (or not) to the surface of the biosensor and plot a binding curve, called a sensogram (see figure 3).
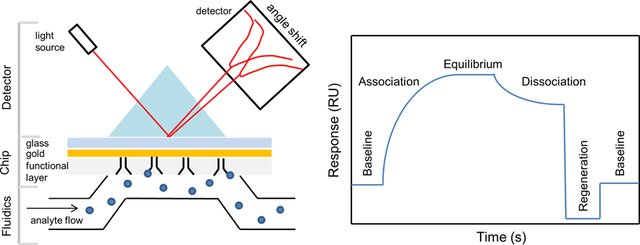
For an animated explanation of SPR, visit https://youtu.be/o8d46ueAwXI.
Hungry for more?
Are you wondering how sensograms differ between samples with and without analyte? Or analytes with high vs. low affinity? Head over to Surface plasmon resonance | Cytiva, formerly GE Healthcare Life Sciences for animated depictions of how to interpret a sensogram in various conditions.
Advantages & Disadvantages of SPR
SPR offers many advantages compared to other biosensing technologies, primarily because of the sensitive, real-time, and quantitative information it yields. Indeed, SPR has very high sensitivity in detecting the presence of analyte via binding/non-binding events (up to a picogram per mm2 of molecules can be detected! This is equivalent to 1/300 of a monolayer of water molecules on a surface. The real-time nature of the measurement enables the measuring of the affinity and the kinetics of the studied molecular interaction, which is crucial in drug development. This information is provided by the sensogram, a type of graph that is made in real-time as the binding reactions occur (see example below). Real-time measurements are very attractive in biological research settings; even more so when they can be done label-free (cf. article “What is a biosensor”), as is the case with SPR. Finally, due to the extremely high sensitivity of SPR, measurements can be done with very small sample sizes (a few microliters are sufficient).
In spite of its many advantages, SPR does have its caveats. The main issue is that SPR only measures the refractive index change on the sensor surface; it is a so-called “integrative sensor”. Imagine a standard scale you would use to weigh yourself: if you put apples and oranges on it, you will not be able to discriminate the weight of the apples from that of the oranges, or be able to count how many of each you have just based on the number given by the scale, as all you get is the total weight. Temperature, medium changes as well as nonspecific binding (i.e. molecules that bind the surface but that we are not interested in detecting) change the refractive index on the surface as well; similarly to the scale problem, we don’t know what exactly we are reading out. SPR is heavily affected by these external influences and requires stabilization. Moreover, it requires long equilibration times, buffers cannot be readily switched during a measurement, and one can only measure molecular interactions in well-defined buffers. In real biological samples, there are a myriad of background molecules in the solution that also bind to the sensor surface (nonspecific binding).
Surely, these non-specific interactions will occur with lower affinity than the specific interactions with analyte of interest. However, in most cases, there are many more non-specific molecules in the testing sample than specific ones (often a million to a billion times more). The non-specific binding therefore completely obscures the binding signal and hampers the limit of detection. The cross-sensitivity problem of SPR towards medium changes, temperature and non-specific binding can be summarized as the environmental noise problem of SPR. Finally, besides SPR’s susceptibility to environmental noise, the angle shifts detected by SPR when molecular binding occurs are minuscule. One therefore needs to measure the angle with a relative precision of 10-5, a precision equivalent to being able to see the Eiffel tower from China… This is only achievable with expensive scientific cameras and equipment.
Did you know?
Although SPR has proven to be a very attractive technology to analyze molecular interactions, its limitations are vast in the context of crude biological samples and non-stabilized measurements. Furthermore, the angle shift of the SPR resonance needs to be measured extremely precisely.
Why is this the case? Why are SPR measurements so challenging? Could it be possible to create a technology that encompasses all the advantages of SPR (label-free, real-time, sensitive, and quantitative), but that could also be used in non-purified samples and better filter out environmental and experimental noise? Stay tuned – The answer to this question will be discussed in our next blog article.
References
Tang, Y., Zeng, X. and Liang, J., 2010. Surface plasmon resonance: an introduction to a surface spectroscopy technique. Journal of chemical education, 87(7), pp.742-746.
Surface plasmon resonance | Cytiva, formerly GE Healthcare Life Sciences
Images
Katharina Kübrich, 2019. Molographic Peptide Arrays: Towards Label-Free Protein Signaturing in Undiluted Blood Plasma. ETH Zürich Master’s Thesis, p. 12.
Tang, Y., Zeng, X. and Liang, J., 2010. Surface plasmon resonance: an introduction to a surface spectroscopy technique. Journal of chemical education, 87(7), pp.742-746.
Damborský, P., Švitel, J. and Katrlík, J., 2016. Optical biosensors. Essays in biochemistry, 60(1), pp.91-100